
What is a Chemical Equation?
A chemical equation is an expression of a chemical process. For example:
In this equation, AgNO3 is mixed with NaCl. The equation shows that the reactants (AgNO3 and NaCl) react through some process (--->) to form the products (AgCl and NaNO3). Since they undergo a chemical process, they are changed fundamentally.
Often chemical equations are written showing the state that each substance is in. The (s) sign means that the compound is a solid. The (l) sign means the substance is a liquid. The (aq) sign stands for aqueous in water and means the compound is dissolved in water. Finally, the (g) sign means that the compound is a gas.
Coefficients are used in all chemical equations to show the relative amounts of each substance present. This amount can represent either the relative number of molecules, or the relative number of moles (described below). If no coefficient is shown, a one (1) is assumed.
On some occasions, a variety of information will be written above or below the arrows. This information, such as a value for temperature, shows what conditions need to be present for a reaction to occur. For example, in the graphic below, the notation above and below the arrows shows that we need a chemical Fe2O3, a temperature of 1000° C, and a pressure of 500 atmospheres for this reaction to occur.
The graphic below works to capture most of the concepts described above:
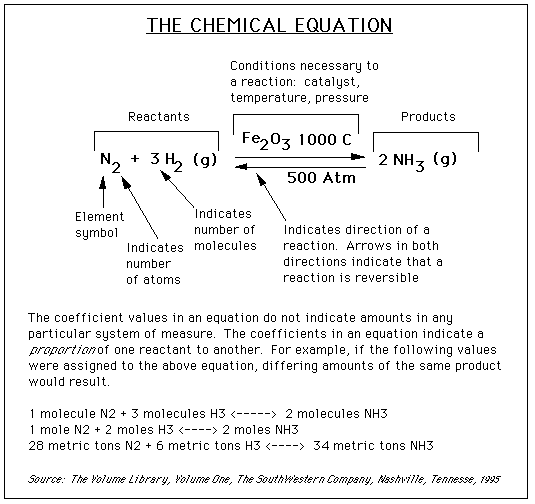
The Mole
Given the equation above, we can tell the number of moles of reactants and products. A mole simply represents Avogadro's number (6.022 x 1023) of molecules. A mole is similar to a term like a dozen. If you have a dozen carrots, you have twelve of them. Similarly, if you have a mole of carrots, you have 6.022 x 1023 carrots. In the equation above there are no numbers in front of the terms, so each coefficient is assumed to be one (1). Thus, you have the same number of moles of AgNO3, NaCl, AgCl, NaNO3. Converting between moles and grams of a substance is often important. This conversion can be easily done when the atomic and/or molecular mass of the substance(s) are known. Given the atomic or molecular mass of a substance, that mass in grams makes a mole of the substance. For example, calcium has an atomic mass of 40 atomic mass units. So, 40 grams of calcium makes one mole, 80 grams makes two moles, etc.Balancing Chemical Equations
+ Fe
Since no coefficients are in front of any of the terms, it is easy to assume that one (1) mole of Al and one (1) mole of Fe3O4 react to form one (1) mole of Al2O3. If this were the case, the reaction would be quite spectacular: an aluminum atom would appear out of nowhere, and two (2) iron atoms and one (1) oxygen atom would magically disappear. We know from the Law of Conservation of Mass (which states that matter can neither be created nor destroyed) that this simply cannot occur. We have to make sure that the number of atoms of each particular element in the reactants equals the number of atoms of that same element in the products. To do this we have to figure out the relative number of molecules of each term expressed by the term's coefficient.
Balancing a simple chemical equation is essentially done by trial and error. There are many different ways and systems of doing this, but for all methods, it is important to know how to count the number of atoms in an equation. For example we will look at the following term.
This term expresses two (2) molecules of Fe3O4. In each molecule of this substance there are three (3) Fe atoms. Therefore in two (2) molecules of the substance there must be six (6) Fe atoms. Similarly there are four (4) oxygen atoms in one (1) molecule of the substance so there must be eight (8) oxygen atoms in two (2) molecules.
Now let's try balancing the equation mentioned earlier:
Developing a strategy can be difficult, but here is one way of approaching a problem like this.
- Count the number of each atom on the reactant and on the product side.
- Determine a term to balance first. When looking at this problem, it appears that the oxygen will be the most difficult to balance so we'll try to balance the oxygen first. The simplest way to balance the oxygen terms is:
Al + 3 Fe3O4---> 4 Al2O3+ Fe Be sure to notice that the subscript times the coefficient will give the number of atoms of that element. On the reactant side, we have a coefficient of three (3) multiplied by a subscript of four (4), giving 12 oxygen atoms. On the product side, we have a coefficient of four (4) multiplied by a subscript of three (3), giving 12 oxygen atoms. Now, the oxygens are balanced.
- Choose another term to balance. We'll choose iron, Fe. Since there are nine (9) iron atoms in the term in which the oxygen is balanced we add a nine (9) coefficient in front of the Fe. We now have:
Al +3 Fe3O4---> 4Al2O3+ 9Fe - Balance the last term. In this case, since we had eight (8) aluminum atoms on the product side we need to have eight (8) on the reactant side so we add an eight (8) in front of the Al term on the reactant side.
Now, we're done, and the balanced equation is:
Percent Composition
There are two types of percent composition problems-- problems in which you are given the formula (or the weight of each part) and asked to calculate the percentage of each element and problems in which you are given the percentages and asked to calculate the formula.
In percent composition problems, there are many possible solutions. It is always possible to double the answer. For example, CH and C2H2 have the same proportions, but they are different compounds. It is standard to give compounds in their simplest form, where the ratio between the elements is as reduced as it can be-- called the empirical formula. When calculating the empirical formula from percent composition, one can convert the percentages to grams. For example, it is usually the easiest to assume you have 100 g so 54.3% would become 54.3 g. Then we can convert the masses to moles; this gives us mole ratios. It is necessary to reduce to whole numbers. A good technique is to divide all the terms by the smallest number of moles. Then the ratio of the moles can be transferred to write the empirical formula.
Example: If a compound is 47.3% C (carbon), 10.6% H (hydrogen) and 42.0% S (sulfur), what is its empirical formula?
To do this problem we need to transfer all of our percents to masses. We assume that we have 100 g of this substance. Then we convert to moles:
Carbon: | 1 | x | 12.01 grams | = 3.94 moles |
Hyrdrogen: | 1 | x | 1.008 grams | = 10.52 moles |
Sulfur: | 1 | x | 32.07 grams | = 1.310 moles |
Now we try to get an even ratio between the elements so we divide by the number of moles of sulfur, because it is the smallest number:
Carbon: | 1.310 | = 3 |
Hydrogen: | 1.310 | = 8 |
Sulfur: | 1.310 | = 1 |
So we have: C3H8 S
Example: Figure out the percentage by mass of hydrogen sulfate, H2SO4.
In this problem we need to first calculate the total mass of the compound by looking at the periodic table. This gives us:
2(1.008) + 32.07 + 4(16.00) g/mol = 98.09 g/mol
Now, we need to take the weight fraction of each element over the total mass (which we just found) and multiply by 100 to get a percentage.
hydrogen: | 98.09 | = | 98.09 | = 0.0206 ∗ 100 = 2.06% |
sulfur: | 98.09 | = 0.327 ∗ 100 = 32.7% | ||
oxygen: | 98.09 | = | 98.09 | = 0.652 ∗ 100 = 65.2% |
Now, we can check that the percentages add up to 100%
So the answer is that H2SO4 is made up of 2.06% H, 32.7% S, and 65.2% O by mass.
Empirical Formula and Molecular Formula
One can calculate the empirical formula from the masses or percentage composition of any compound. We have already discussed percent composition in the section above. If we only have mass, all we are doing is essentially eliminating the step of converting from percentage to mass.
Example: Calculate the empirical formula for a compound that has 43.7 g P (phosphorus) and 56.3 grams of oxygen. First we convert to moles:
1 | x | 30.97 grams | = 1.41 moles | ||
1 | x | 16.00 grams | = 3.52 moles |
Next we divide the moles to try to get an even ratio.
Phosphorus: | 1.41 | = 1.00 |
Oxygen: | 1.41 | = 2.50 |
When we divide, we did not get whole numbers so we must multiply by two (2). The answer = P2O5
Calculating the molecular formula once we have the empirical formula is easy. If we know the empirical formula of a compound, all we need to do is divide the molecular mass of the compound by the mass of the empirical formula. It is also possible to do this with one of the elements in the formula; simply divide the mass of that element in one mole of compound by the mass of that element in the empirical formula. The result should always be a natural number.
Example: if we know that the empirical formula of a compound is HCN and we are told that a 2.016 grams of hydrogen are necessary to make the compound, what is the molecular formula? In the empirical formula hydrogen weighs 1.008 grams. Dividing 2.016 by 1.008 we see that the amount of hydrogen needed is twice as much. Therefore the empirical formula needs to be increased by a factor of two (2). The answer is:
H2C2N2.
Density
Concentrations of Solutions
The concentration of a solution is typically given in molarity. Molarity is defined as the number of moles of solute (what is actually dissolved in the solution) divided by the volume in liters of solution (the total volume of what is dissolved and what it has been dissolved in).
Molarity = | liters of solution |
Molarity is probably the most commonly used term because measuring a volume of liquid is a fairly easy thing to do.
Example: If 5.00 g of NaOH are dissolved in 5000 mL of water, what is the molarity of the solution?
One of our first steps is to convert the amount of NaOH given in grams into moles:
1 | x | (22.9 + 16.00 + 1.008)g | = 0.125 moles |
Now we simply use the definition of molarity: moles/liters to get the answer
Molarity = | 5.00 L of soln | = 0.025 mol/L |
So the molarity (M) of the solution is 0.025 mol/L.
Molality is another common measurement of concentration. Molality is defined as moles of solute divided by kilograms of solvent (the substance in which it is dissolved, like water).
Molality = | kg of solvent |
Molality is sometimes used in place of molarity at extreme temperatures because the volume can contract or expand.
Example: If the molality of a solution of C2H5OH dissolved in water is 1.5 and the mass of the water is 11.7 kg, figure out how much C2H5OH must have been added in grams to the solution.
Our first step is to substitute what we know into the equation. Then we try to solve for what we don't know: moles of solute. Once we know the moles of solute we can look at the periodic table and figure out the conversion from moles to grams.
|
Now we simply use the definition of molarity: moles/liters to get the answer
| |||||
| |||||
| |||||
|
It is possible to convert between molarity and molality. The only information needed is density.
Example: If the molarity of a solution is 0.30 M, calculate the molality of the solution knowing that the density is 3.25 g/mL.
To do this problem we can assume one (1) liter of solution to make the numbers easier. We need to get from the molarity units of mol/L to the molality units of mol/kg. We work the problem as follows, remembering that there are 1000 mL in a Liter and 1000 grams in a kg. This conversion will only be accurate at small molarities and molalities.
1 L | x | 3.25 g | x | 1000 mL | x | 1 kg | = 0.09 mols / kg |
It is also possible to calculate colligative properties, such as boiling point depression, using molality. The equation for temperature depression or expansion is
Where:
ΔT is temperature depression (for freezing point) or temperature expansion (for boiling point) (°C)
Kf is the freezing point constant (kg °C/mol)
m is molality in mol/kg
Example: If the freezing point of the salt water put on roads is -5.2° C, what is the molality of the solution? (The Kf for water is 1.86 °C/m.)
This is a simple problem where we just plug in numbers into the equation. One piece of information we do have to know is that water usually freezes at 0° C.
ΔT = Kf * m
ΔT/Kf = m
m = 5.2/1.86
m = 2.8 mols/kg